Games To Teach Genetic Engineering
Purpose: One of the purposes of this lesson is to pique the students’ interest in genetic engineering and technology by showing them a few interesting examples of genetic engineering using organisms that they are familiar with (chicken, apples & salmon). Another purpose of this lesson is to connect genetics with what students have already learned about DNA replication, transcription, and translation and formatively assess their understanding of genetic engineering techniques. Objectives:. Given an unfamiliar genetic engineering experiment, students will be able to identify and sequence the steps in a genetic engineering and relate them to the more general processes involved in all genetic engineering experiments. Resources:.
Your students should have opportunities to analyze and evaluate the processes and impacts of genetic engineering. The following activities will help. Lesson for Kids.
Computer/projector for powerpoint presentation and the “Harvest of Fear” online genetic engineering experiment. Powerpoint presentation slides for the students. Recombinant DNA Laboratory. Yellow plasmid DNA template. Green 'gene of interest' DNA template. Purple restriction enzyme template. Genetic engineering flipbook description and template.
Colored pencils (1 set per table). Scissors and scotch tape Instructional Sequence:. When the students come in, I will have them draw from a hat to determine the genetic disorders that they will be studying for their projects. Have students come to the front of the room to pick up the powerpoint slides/genetic disorder task description. Have the students read through the genetic disorder assignment description quietly. Describe the assignment out-loud and then show students the wikipage using the projector screen. Begin the powerpoint presentation with students following along with the slides in front of them.
Questions to be asked during the powerpoint presentation:. What do you think that genetic engineering is used for?. Why are bacterial plasmids used as vectors?. When is DNA ligase used in DNA replication?. How can we determine that the foreign DNA has been taken up by the host cell’s DNA?. Why might genetic engineering be a useful technique?.
Navigate to the PBS “Harvest of Fear” webpage and complete the “Engineer a crop” simulation. Ask students leading questions about each step in the process of engineering the crop such as:. Why are we doing this?. What purpose does this serve?. Why are we using this organism for this particular step in the experiment?. What is our end product and how do we know that our experiment was successful?. Have students work to complete the paper recombinant DNA laboratory in cooperative learning groups.
Explore A Cell
Emphasize that this simulation illustrates the first two steps of a genetic engineering experiment when recombinant DNA is created. Go over analysis questions as a class. Allow students to begin work on the genetic engineering flipbook. Genetic Engineering Coloring Flipbook In this activity you will be creating a flipbook to show the stages of a genetic engineering experiment in which a human gene sequence containing the insulin gene is inserted into a bacterial plasmid.
Diagrams of each step have been provided for you. You will need to match the six steps listed below with the correct image on your flipbook sheet. The images are not drawn in the correct sequence on your flipbook sheet.
Number and write each step title on the back of its matching flipbook card and provide a brief description of the step. You may use your powerpoint notes from Friday and pages 227-228 in your textbook to help you create these descriptions. Step #1 = Identify the gene of interest (insulin gene) and the vector (plasmid). Step #2 = Cut DNA with restriction enzyme. Step #3 = Create recombinant DNA. Step #4 = Host cells take up recombinant DNA.
Step #5 = Cloning. Step #6 = Screening Once you have identified and provided brief descriptions of each step, label and color each of the following structures on your cards. A few of these structures will only be present in one or two steps. Gene of interest (insulin gene) = blue. Plasmid DNA = green. Bacteria cell(s) = red.
Bacterial DNA = orange. Complete human chromosome = yellow. Sticky ends = pink. DNA ligase = purple.
Summary Students construct paper recombinant plasmids to simulate the methods genetic engineers use to create modified bacteria. They learn what role enzymes, DNA and genes play in the modification of organisms. For the particular model they work on, they isolate a mammal insulin gene and combine it with a bacteria's gene sequence (plasmid DNA) for production of the protein insulin. This engineering curriculum meets Next Generation Science Standards (NGSS). Engineering Connection Bacteria are the most common organisms modified by genetic engineers due to the simple structures of bacteria cells compared to those of eukaryotic cells.
Engineers are able to add genes to bacteria using recombinant plasmids, which enable the bacteria to produce the desired beneficial proteins. Students use a paper model to simulate this real-life process used by bio-technicians.
Pre-Req Knowledge A basic understanding of protein synthesis and DNA's role in the cell/body is helpful so students can follow how changes in DNA result in major changes in the characteristics of organisms. Learning Objectives After this activity, students should be able to:. Model and describe the process used by engineers to modify the genome of bacteria. Explain why bacteria are genetically modified more often than other organisms. Discuss possible applications for genetically modified bacteria. More Curriculum Like This. Each TeachEngineering lesson or activity is correlated to one or more K-12 science, technology, engineering or math (STEM) educational standards.
All 100,000+ K-12 STEM standards covered in TeachEngineering are collected, maintained and packaged by the Achievement Standards Network (ASN), a project of D2L (www.achievementstandards.org). In the ASN, standards are hierarchically structured: first by source; e.g., by state; within source by type; e.g., science or mathematics; within type by subtype, then by grade, etc. Medical technologies include prevention and rehabilitation, vaccines and pharmaceuticals, medical and surgical procedures, genetic engineering, and the systems within which health is protected and maintained. (Grades 9 - 12) Do you agree with this alignment? Yes No Thanks for your feedback!.
The sciences of biochemistry and molecular biology have made it possible to manipulate the genetic information found in living creatures. (Grades 9 - 12) Do you agree with this alignment? Yes No Thanks for your feedback! Materials List Each group needs:. scissors. tape, 3 small pieces., one per group; these represent plasmid DNA and mammal DNA containing the insulin gene; helpful to print page 1 on different colored paper than page 2., one per group., one per student. stapler (can be shared among groups) Introduction/Motivation How big are bacteria?
(Answer: most are only a few micrometers. Micrometers are one millionth of a meter. 1 cm = 10,000 micrometers; 1 mm = 1,000 micrometers) Bacteria are so small that most are less than one-tenth of the diameter of a human hair. How many different species of bacteria exist? (Answer: Estimates vary from 10 million to 1 billion species.) So far, we have only discovered less than 10,000 different species, but some scientists estimate that as many as 1 billion different species of bacteria may exist on Earth! How prolific are bacteria? Bacteria are so plentiful that a 20-ounce bottle of water may contain up to 600 million bacteria!
Bacteria are everywhere, and most of the time they are harmless. In fact, many are beneficial to people because they are useful and necessary to a healthy human body and environment. What are some examples of these 'good' bacteria? (Possible answers: E.coli within the intestines of mammals, bacteria within the soil, bacteria used to make foods such as yogurt.) Without bacteria, we would not be able to digest food or produce some of our favorite foods such as yogurt and cheese. The bacteria we might generally call 'bad' for us include those responsible for causing illnesses like food poisoning. Do you think genetic engineers could use and modify bacteria for any purpose? (Answer: Yes) Bacteria are the most commonly modified organisms.
Let's look at how we can modify these bacteria and why we would want to modify them. Vocabulary/Definitions Acronym for deoxyribonucleic acid, which is a molecule that contains an organism's complete genetic information. The molecular unit of an organism that contains information for a specific trait (specific DNA sequence). An entire set of genes for an organism. (noun) A representation of something for imitation, comparison or analysis, sometimes on a different scale. (verb) To make something to help learn about something else that cannot be directly observed or experimented upon. The circular DNA structure used by bacteria.
A cell without a nucleus and membrane-bound organelles. DNA to which a section has been removed and replaced (recombined) with a new sequence. An enzyme that 'cuts' DNA when specific base pair sequences are present.
Procedure Background Bacteria can be adapted to produce a number of useful materials. Because of the simple structures of bacterial cells, they are the most commonly modified organisms.
Many times, and in this activity, a gene is simply added to the bacteria, causing the bacteria to be able to produce a useful protein, such as insulin. Other changes to bacteria can reconfigure the cellular respiration product to create desirable byproducts such as diesel or plastic molecules instead of the usual byproduct, such as carbon dioxide. The common method used for genetically modifying bacteria is to use recombinant plasmids. Plasmids are circular pieces of DNA; when placed near bacteria, the plasmid is absorbed and incorporated into the bacterial cell. Once inside the bacteria, the plasmid is treated the same as the bacteria's original DNA. This means that the bacteria will use this new DNA from the plasmid to create proteins, and the plasmid will be replicated when the cell divides. The process of creating genetically modified bacteria used in this activity is one of the simplest methods.
First, a desired gene must be selected from some (any) organism, that is, the gene that codes for the creation of insulin protein, and removed from the DNA of that organism. Genes are removed using restriction enzymes. These enzymes search for specific nucleotide sequences in the DNA, called recognition sites, where they 'cut' the DNA by breaking certain bonds. When the bonds are broken in a staggered manner it creates 'sticky ends.' If the enzyme breaks the bond to create a straight cut, the ends are called 'blunt ends.' The next step is to use the same restriction enzyme to cut open the plasmid. The isolated gene is now placed where the plasmid was cut, and they are bonded together using another enzyme called ligase.
Reporting for the.NET Framework The award-winning banded report designer and viewer for WinForms, ASP.NET, Silverlight and WPF. Windows 8 XAML Next-Gen.NET Tools The new DevExpress Windows® 8 XAML Subscription helps you create compelling touch-enabled business solutions that leverage the best parts of Windows® 8 when targeting next generation devices such as Microsoft Surface.
Now a recombinant plasmid has been produced. The final step is to get the plasmid into a bacteria cell. Sometimes simply placing the bacteria in the correct environment is enough to artificially induce the bacteria to intake the recombinant DNA, otherwise some special method may be required if the plasmid is too large to cross the bacteria's cell membrane. Figure 1.shows a diagram of the entire process. The process to build a recombinant plasmid to modify bacteria. Copyright © 2010 Darryl Leja, National Human Genome Research Institute, U.S.
National Institutes of Health Before the Activity. Gather materials and make copies of the, one per group, and, one per person. Make copies of the, one per group; it is helpful if the plasmid DNAs (page 1) are printed on different colored paper from the mammal DNAs (page 2) to help distinguish them during the activity.
Then cut the DNA sequences into strips. If time is short, tape the cut-out plasmid DNA into circles with the DNA sequence facing outward. Otherwise, have students do this in step 2.
With the Students. Divide the class into groups of two students each. Hand out to each group a worksheet and its two strips of DNA sequences, pointing out which will be used to create the plasmid, and which is the mammal DNA containing the insulin gene. Direct groups to tape together the ends of the plasmid DNA to form a circular piece of DNA (see Figure 2) so that the printed sequence is visible on the outside of the plasmid. This is the initial plasmid that will be modified with the insulin gene. Illustration of the circular DNA created in step 2. Copyright © 2013 Matthew Zelisko, GK-12 Program, University of Houston.
Find the recognition sites on both the plasmid and the mammal DNA. The restriction enzyme being used will search for a specific base pair sequence on the DNA to cut. This sequence and the cut pattern are shown in Figure 3. Have students look for this sequence on both DNA strands.
Anywhere they find the full sequence is a recognition site; have them draw a dotted line at the site where the restriction enzyme will cut. Doing this usually prevents students from cutting straight across in the next step. A restriction enzyme recognition site with dashed line showing where the enzyme will break apart the DNA. Copyright © 2013 Matthew Zelisko, GK-12 Program, University of Houston.
Have students apply the restriction enzyme (represented by scissors in this model) to cut the DNA at the marked locations. Performed correctly, students make one cut through the plasmid and two cuts on the DNA containing the insulin gene. These cuts are made on either side of the designated gene. They are 'staggered' cuts, forming the 'sticky ends.' Now that the desired gene is isolated, it is ready to be added to the plasmid DNA (see Figure 4).
Fight The Bad Bacteria
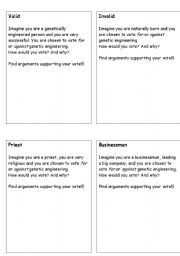
Fun Ways To Teach Genetic Engineering
In blue, the model plasmid after being cut by a restriction enzyme. In red, the mammal DNA with cuts and its isolated insulin gene with sticky ends. Copyright © 2013 Matthew Zelisko, GK-12 Program, University of Houston. Next, have students use the ligase enzyme (represented by tape in this model) to rebuild the plasmid with the new gene incorporated. If the DNA sequences have been correctly cut, the base pairs from each end of the gene match exactly with the cuts made in the plasmid.
Tape the ends of the gene to the matching sticky end on the plasmid (see Figure 5). The final recombinant model plasmid, a merger of the original plasmid DNA and the added insulin gene. Assessment Pre-Activity Assessment Review: Quickly do a review of bacteria, emphasizing bacteria structure. Discuss the pervasiveness of bacteria in everyday life and some roles they play, both beneficial (digestive health) and harmful (illness). Alternatively, print out the Lesson Background section and have students read through it.
Activity Embedded Assessment Building a Model Recombinant Plasmid: Direct student pairs to apply their understanding of genetics to modify plasmids. After finishing the modification, have teams complete the to demonstrate their understanding of the modification process. Post-Activity Assessment Questions: Administer the.
Use the to individually gauge students' understanding of the genetic modification of bacteria and its potential benefits and dangers. Updated December 9, 2013.
Wikipedia, The Free Encyclopedia. Accessed December 10, 2013. Contributors Matthew Zelisko, Kimberly Anderson Copyright © 2013 by Regents of the University of Colorado; original © 2013 University of Houston Supporting Program National Science Foundation GK-12 and Research Experience for Teachers (RET) Programs, University of Houston Acknowledgements Acknowledgements This digital library content was developed by the University of Houston's College of Engineering under National Science Foundation GK-12 grant number DGE 0840889. However, these contents do not necessarily represent the policies of the NSF and you should not assume endorsement by the federal government. Last modified: January 30, 2018.